文章信息
- 刘丹, 张帅, 唐玉姣, 尹静, 谭兴伶, 陈茜, 于志国, 林俊杰
- LIU Dan, ZHANG Shuai, TANG Yu-jiao, YIN Jing, TAN Xing-ling, CHEN Xi, YU Zhi-guo, LIN Jun-jie
- 三峡支流消落带表层沉积物氮矿化动力学参数估算
- Parameter estimates of sediment nitrogen mineralization kinetics in the water level fluctuation zone of a Three Gorges Reservoir tributary
- 农业环境科学学报, 2018, 37(4): 766-773
- Journal of Agro-Environment Science, 2018, 37(4): 766-773
- http://dx.doi.org/10.11654/jaes.2017-1482
文章历史
- 收稿日期: 2017-09-29
- 录用日期: 2017-11-27
2. 重庆三峡学院三峡库区水环境演变与污染防治重庆高校市级重点实验室, 重庆 万州 404155;
3. 南京信息工程大学水文气象学院, 南京 210044
2. Key Laboratory of Water Environment Evolution and Pollution Control in Three Gorges Reservoir, Chongqing Three Georges University, Wanzhou 404155, China;
3. College of Hydrometeorology, Nanjing University of Information Science and Technology, Nanjing 210044, China
土壤氮素主要以有机氮形态存在,是无机氮的重要来源,其矿化特征对植物养分供给具有重要意义,但其需经微生物转化成铵态氮和硝态氮才能被植物直接利用[1]。而无机氮极易淋溶进入水体,从而增加水体富营养化风险。
特殊的调蓄水制度使三峡支流不同水位高程消落带经历了不同程度的干湿循环[2-3],消落带生源要素周转速率、氧化还原状态、水土界面过程等均发生了明显变化[4-5]。三峡支流蓄水期水流缓慢[6],氮素进入水体不易迁移扩散,可能造成水体富营养化等水生态问题,因此,查明干湿循环条件下,三峡支流消落带沉积物氮矿化动力学过程至关重要。
氮矿化是陆地生态系统氮循环的关键[7],主要受凋落物输入、微生物和酶活性以及根际过程等生物因子[8-10],以及温度、湿度、pH等非生物因素的影响[11-14]。氮矿化动力学参数可用来衡量氮矿化潜力和供氮能力,通过模型拟合可进行动力学参数估算。氮矿化模型按有机物分解过程可分为零阶、一阶、双一阶和混阶动力学模型[15-17];按建模方式可分为有效积温模型(EATM)、机理模型和功能模型[18];按有机氮性质分为One-pool、Two-pool、Special及多氮库模型[19]。张玉玲等[20]研究表明,Special模型是长期施肥水稻土氮矿化过程的最佳模型;卢红玲等[21]研究黄土高原石灰性土壤氮矿化模型发现,Two-pool和Special模型优于One-pool和有效积温模型,且Special模型淹水条件下更优;Gil等[22]研究发现,Special模型更适合长期施肥后土壤氮矿化过程模拟;Li等[23]研究上海地区水稻土壤氮矿化模型发现,Two-pool和Special模型对氮矿化的过程模拟效果最好,且Special模型的参数最优;Camargo等[24]对巴西南部土壤氮矿化过程进行模型拟合,发现One-pool和Two-pool模型拟合效果较好,但参数估算过程较为复杂;刘青丽等[25]研究表明,在变温条件下有效积温模型能更好地模拟土壤氮矿化过程,而指数模型能较好地描述氮矿化对水分变化的响应。可见,查明不同环境条件下氮矿化动力学最佳模型至关重要。
本研究以三峡支流彭溪河消落带为研究对象,从有机氮分解过程角度结合One-pool、Two-pool、Special、EATM对消落带沉积物氮矿化过程进行了拟合,通过多元回归建立了基本理化性质与拟合参数的估算方程,旨在为查明三峡支流水体富营养化频发和消落带植被适生性下降等问题提供科学依据。
1 材料与方法 1.1 研究区域与样品采集三峡库区特殊的调蓄水制度使得库区水位在145~175 m之间呈年际周期性涨落。本研究于2015年6月库区水位最低、消落带裸露期间采集三峡支流澎溪河上游(渠口镇)和下游(双江镇)两个水文断面的低水位(150 m)和高水位(170 m)高程的表层(0~15 cm)沉积物样品,每个水位高程由3个随机采样点组成(图 1)。采集的原状新鲜样品于4 ℃保存,一部分用于氮矿化培养实验,另一部分经冻干、剔除植物根系、过筛后用于基本理化性质测定。
![]() |
图 1 澎溪河消落带采样点位 Figure 1 Sampling sites in the water level fluctuating zone of Pengxi River |
2013—2017年三峡库区万州水文站水位波动和水位高程与淹水时间的关系见图 2。消落带水位高程和淹水时间呈显著负相关,水位越低淹水时间越长,150 m水位高程淹水时间约325 d·a-1,主要集中在8月至次年6月,170 m水位高程主要淹水时间约123 d·a-1,主要集中10月至次年1月。
![]() |
图 2 水位高程与淹水时间之间的关系 Figure 2 Relationship of water level altitude with flood time |
采用连续淹水厌氧培养法对氮矿化速率进行了测定[26],具体步骤如下:准确称取10.00 g经预处理样品于50 mL培养瓶,按水土比2:1加入去离子水,以高纯氮(>99.99%)保持厌氧,控制氧气 < 1×10-6,使体系始终处于厌氧状态,密封,每个样品3次重复,于35 ℃恒温培养箱中避光培养[27]。分别于第3、7、14、21、28 d破坏性取样,因厌氧条件,故不考虑NO3--N变化,只测定NH4+-N含量[28]。
1.4 分析方法pH值采用0.01 mol·L-1 CaCl2浸提法测定,有机质(OM)采用重铬酸钾容量法测定,NH4+-N采用靛酚蓝比色法测定,NO3--N采用2 mol·L-1 KCl浸提比色法测定,沉积物粒径组成采用比重法测定,总磷(P)采用碱熔-钼锑抗分光光度法测定,总碳(C)和总氮(N)用元素分析仪(意大利EA3000)测定,溶解性有机碳(DOC)用总有机碳分析仪测定(TOC-VCPN)。
1.5 氮矿化模型 1.5.1 One-pool模型One-pool模型是在一阶指数模型基础上提出的,假设氮库由单一组分组成,具体如下[29]:

式中:Nm为累积氮矿化量,mg·kg-1;N为总氮含量,g·kg-1;fd为易矿化氮占总氮比值,%;kd为易矿化氮矿化速率常数,d-1。
1.5.2 Two-pool模型将有机氮库分为两部分,即易矿化氮库和难矿化氮库,具体如下[30]:

式中:kr为难矿化氮矿化速率常数,d-1。
1.5.3 Special模型Special模型是在双库氮矿化模型基础上提出的,假设氮库存在一个较稳定且较慢矿化的部分,且该部分更符合零阶方程,具体如下[31]:

式中:kt为较慢矿化部分矿化速率常数,d-1。
1.5.4 有效积温模型(EATM)有效积温模型是以温度为主导因素的模型,具体如下[32]:

式中:T为培养温度,℃;T0为基点温度,℃;t为培养时间,d;k和n为矿化常数。n<1时,单位有效积温所矿化氮量随培养时间的增加逐渐减少;n>1时相反。
1.6 模型评价与分析利用Microsoft Excel 2010对数据进行处理,利用SigmaPlot 12.0对四种氮库模型进行拟合及绘图,利用IBM SPSS Statistic 20对数据进行统计分析,并通过多元逐步回归分析建立基于沉积物基本理化性质的氮矿化动力学参数估测方程,用调整的确定系数和均方根误差判断模型优劣。
模型调整的确定系数(Radj2)公式为:

式中:n指观测样品数;R2为模型确定系数;M为变量个数,理论上Radj2位于0~1之间,其越接近1,表明模型模拟越准确。
均方根误差(RMSE)公式为:
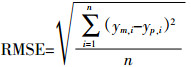
式中:ym指观测值;yp指估测值。RMSE越小,表明预测误差越小,模型精度越高。
2 结果与分析 2.1 消落带沉积物基本理化性质沉积物基本理化性质见表 1。从表 1可知,研究区沉积物C、N、C/N、OM在低水位高程含量更高,而P与之相反,DOC在水位高程分布上无显著差异。沉积物粘粒和粉粒在水位高程上分布表现为低水位高程>高水位高程,而砂砾与之相反。总体上,砂砾>粉粒>粘粒。NH4+-N表现为低水位高程>高水位高程,而NO3--N分布与其相反。
对三峡支流消落带沉积物氮矿化过程采用One-pool、Two-pool、Special及有效积温模型进行拟合见图 3,模型参数见表 2。在水位高程上,净氮矿化累积量均表现为低水位高程大于高水位高程,且随时间延长显著增加(P<0.05);有效积温模型对不同水位高程沉积物矿化情况进行拟合得到的n值均小于1,表明单位有效积温所矿化氮量随培养时间的增加逐渐减少。k值代表矿化强度,相关分析表明,k值与累积矿化氮呈显著正相关。One-pool模型在低水位高程Nd值最大,RMSE值最低,Special模型在高水位高程Nd值最大,RMSE值最低。
![]() |
图 3 沉积物氮矿化模型拟合 Figure 3 Model fitting of soil nitrogen mineralization kinetics |
将氮矿化模型拟合参数与沉积物基本理化性质进行相关分析(表 3),结果表明,fd与C、N、C/N、NH4+-N、OM、粉粒呈极显著负相关(P<0.01),与砂砾、NO3--N呈极显著正相关(P<0.01);kd与C、N、C/N、NH4+-N、OM、粉粒呈极显著正相关(P<0.01),与砂砾、NO3--N呈极显著负相关(P<0.01)。可见,C、N、C/N、OM、NH4+-N、NO3--N、粉粒和砂砾可能为预测沉积物氮矿化动力学参数的决定因子。
![]() |
将模型参数fd和kd作为因变量,利用相关性分析所得预测沉积物氮矿化动力学参数的决定因子(C、N、C/N、OM、NH4+-N、NO3--N、粉粒和砂砾)作为自变量进行多元逐步线性回归,建立的模型参数预测方程见表 4。从表 4可知,氮矿化动力学模型参数fd和kd可用C/N和OM进行估算,模型参数fd和kd的Radj2分别为0.985和0.963,RMSE分别为0.006和0.000 4,P值均小于0.01。可见,该预测方程可较好地预测消落带氮矿化模型参数fd和kd。
澎溪河消落带沉积物C、N、C/N、NH4+-N、NO3--N含量随水位高程变化差异显著(表 1),其中,C、N、C/N和NH4+-N随水位高程降低而增加,而NO3--N含量随水位高程降低而降低。可能原因为,一方面,低水位高程淹水胁迫时间较长(年淹水时间为325 d),缺氧条件下NH4+-N向NO3--N转化受限,且NH4+-N带正电荷,易被沉积物吸附,不易流失,表现为NH4+-N累积[33];另外,沉积物NO3--N带负电荷溶水性,使其更易进入水体[34-35],表现为NO3--N流失;同时,还原条件下沉积物碳氮矿化较慢,可能是导致低水位高程C、N和C/N较高的原因。另一方面,高水位高程淹水时间较短(年淹水时间为123 d),落干条件下沉积物暴露于空气中,NH4+-N易通过硝化作用转化为NO3--N,且可通过NH3形式挥发而损失[36-38],表现为NH4+-N流失、NO3--N累积。而植被适生性、多样性和生物量等均随高程增加而增强(大)[39],可能与沉积物NH4+-N和NO3--N分布存在一定内在联系。另外,沉积物氮矿化累积量培养前期快速上升,后期趋于稳定,这与顾春朝等[40]所得结果一致。可能原因为,一方面,淹水初期,厌氧微生物迅速繁殖并将有机氮分解为铵态氮。随着培养时间延长,铵态氮积累,厌氧微生物数量饱和。李建兵等[41]研究表明,过高的铵态氮可能抑制微生物生长,使氮矿化累积量趋于稳定。另一方面,短期培养过程中氮矿化主要来自易分解氮库,这部分氮库受团聚体等物理化学保护较弱,易被优先分解矿化。随着培养时间延长易分解氮消耗殆尽,而沉积物中难分解氮库组分受团聚体等物理化学作用保护较强,难于分解矿化[42-43]。
四种氮矿化动力学模型均能够较好拟合消落带沉积物氮矿化动力学过程,其中One-pool模型对低水位高程拟合RMSE值最小,效果最好;Special模型对高水位高程拟合RMSE值最小,效果最好。消落带沉积物氮素矿化过程中,不同水位高程沉积物易矿化氮库矿化势(Nd)存在显著差异(表 2),表现为在低水位高程高于高水位高程,沉积物C、N、C/N、NH4+-N、NO3--N、OM、粉粒、砂砾与沉积物易矿化氮库矿化势(fd)和易矿化速率(kd)显著相关,受沉积物理化性质影响较大。刘杏认等[44]研究表明,在一定湿度范围内含水量增加使沉积物氮矿化速率增加。Harrison-Kirk等[45]的研究表明土壤质地会影响土壤含水量和通气孔隙,从而影响氮矿化过程。Hanan等[13]的研究表明pH的变化会对氮矿化过程产生影响。林俊杰等[46]研究表明,消落带沉积物氨化、硝化及净氮矿化速率与其N本底值正相关。氮矿化动力学参数估算表明,C/N和OM是控制模型参数fd和kd的关键因素。Haer等[47]研究表明,OM含量和粘粒比例是影响印度耕地沉积物氮矿化动力学参数估算的主要因素;Schomberg等[48]的研究表明C和N是预测土壤氮矿化潜力的主要因素;周吉利等[49]研究表明,微生物量碳和pH值决定了中亚热带红壤区沉积物的氮矿化过程。此外,本研究尚未考虑季节性温度升高与干湿循环耦合关系对消落带表层沉积物氮矿化动力学过程的影响,在未来的工作中需进一步研究。
4 结论干湿循环加速了消落带氮矿化动力学过程,增加了低水位高程消落带沉积物易矿化氮重新淹水后大量进入水体的风险,One-pool模型和Special模型分别是低水位和高水位高程氮矿化动力学拟合的最佳模型,其动力学参数与沉积物C、N、NH4+-N、NO3--N、OM、C/N、粉粒和砂砾显著相关;且C/N和OM可用于氮矿化动力学模型参数估算,对深入理解三峡支流消落带沉积物氮矿化机制与消落带植被适生性下降、水体富养化之间的关系具有指示意义。
[1] |
白日军, 杨治平, 张强, 等. 晋西北不同年限小叶锦鸡儿灌丛土壤氮矿化和硝化作用[J]. 生态学报, 2016, 36(24): 8008-8014. BAI Ri-jun, YANG Zhi-ping, ZHANG Qiang, et al. Soil nitrogen mineralization and nitrification under Caragana microphylla shrubs of different ages in the northwestern Shanxi Loess Plateau[J]. Acta Ecologica Sinica, 2016, 36(24): 8008-8014. |
[2] |
Wang Y J, Chen F Q, Zhang M, et al. The effects of the reverse seasonal flooding on soil texture within the hydro-fluctuation belt in the Three Gorges Reservoir, China[J]. Journal of Soils & Sediments, 2018, 18(1): 109-115. |
[3] |
林俊杰, 张帅, 杨振宇, 等. 干湿循环对三峡支流消落带沉积物中可转化态氮及其形态分布的影响[J]. 环境科学, 2015, 36(27): 2460-2463. LIN Jun-jie, ZHANG Shuai, YANG Zhen-yu, et al. Effect of drought and subsequent re-wetting cycles on transferable nitrogen and its form distribution in the sediment of water level fluctuating zone in the tributary of Three Gorge Reservoir Areas[J]. Environmental Science, 2015, 36(27): 2460-2463. |
[4] |
赫斌, 李哲, 冯婧, 等. 三峡澎溪河高阳平湖高水位期间磷-藻生态模型研究[J]. 湖泊科学, 2016, 28(6): 1244-1255. HE Bin, LI Zhe, FENG Jing, et al. Study on the phosphorus-algal ecological modelling during high water level period in Lake Gaoyang of Pengxi River, Three Gorges Reservoir[J]. J Lake Sci, 2016, 28(6): 1244-1255. DOI:10.18307/2016.0610 |
[5] |
胥焘, 王飞, 郭强, 等. 三峡库区香溪河消落带及库岸土壤重金属迁移特征及来源分析[J]. 环境科学, 2014, 35(4): 1502-1508. XU Tao, WANG Fei, GUO Qiang, et al. Transfer characteristic and source identification of soil heavy metals from water-level-fluctuating zone along Xiangxi River, Three-Gorges Reservoir Area[J]. Environmental Science, 2014, 35(4): 1502-1508. |
[6] |
付莉, 张磊, 蔚建军, 等. 三峡库区支流回水区水体分层与藻类生长[J]. 环境工程学报, 2015, 9(5): 2265-2271. FU Li, ZHANG Lei, WEI Jian-jun, et al. Water stratification and its relevance to growth of algal community at backwater area in Three Gorges Reservoir[J]. Chinese Journal of Environmental Engineering, 2015, 9(5): 2265-2271. DOI:10.12030/j.cjee.20150539 |
[7] |
赵丽, 王书航, 姜霞, 等. 蠡湖表层沉积物氮矿化过程及其赋存形态变化[J]. 环境科学, 2016, 37(12): 4626-4632. ZHAO Li, WANG Shu-hang, JIANG Xia, et al. Variation of nitrogen forms in sediments of Lihu Lake during mineralization[J]. Environmental Science, 2016, 37(12): 4626-4632. |
[8] |
Bai J H, Gao H F, Xiao R, et al. A review of soil nitrogen mineralization as affected by water and salt in coastal wetlands:Issues and methods[J]. Clean-Soil Air Water, 2012, 40(10): 1099-1105. DOI:10.1002/clen.201200055 |
[9] |
Carrillo Y, Ball B A, Molina M. Stoichiometric linkages between plant litter, trophic interactions and nitrogen mineralization across the litter-soil interface[J]. Soil Biology & Biochemistry, 2016, 92: 102-110. |
[10] |
Deressa A. Effects of soil moisture and temperature on carbon and nitrogen mineralization in grassland soils fertilized with improved cattle slurry manure with and without manure additive[J]. Physical Review E Statistical Nonlinear & Soft Matter Physics, 2015, 2(1): 1-9. |
[11] |
葛晓敏, 王瑞华, 唐罗忠, 等. 不同温湿度条件下杨树人工林土壤氮矿化特征研究[J]. 中国农学通报, 2015, 31(10): 208-213. GE Xiao-min, WANG Rui-hua, TANG Luo-zhong, et al. Study on the effects of temperature and moisture on nitrogen mineralization of soil in poplar plantations[J]. Chinese Agricultural Science Bulletin, 2015, 31(10): 208-213. DOI:10.11924/j.issn.1000-6850.casb14110061 |
[12] |
刘碧荣, 王常慧, 张丽华, 等. 氮素添加和刈割对内蒙古弃耕草地土壤氮矿化的影响[J]. 生态学报, 2015, 35(19): 6335-6343. LIU Bi-rong, WANG Chang-hui, ZHANG Li-hua, et al. Effect of nitrogen addition and mowing on soil nitrogen mineralization in abandoned grasslands in Inner Mongolia[J]. Acta Ecologica Sinica, 2015, 35(19): 6335-6343. |
[13] |
Hanan E J, Schimel J P, Dowdy K, et al. Effects of substrate supply, pH, and char on net nitrogen mineralization and nitrification along a wildfire-structured age gradient in chaparral[J]. Soil Biology & Biochemistry, 2016, 95: 87-99. |
[14] |
Liu Y, He N P, Wen X F, et al. Patterns and regulating mechanisms of soil nitrogen mineralization and temperature sensitivity in Chinese terrestrial ecosystems[J]. Agriculture Ecosystems & Environment, 2016, 215: 40-46. |
[15] |
Li H L, Han Y, Cai Z C. Nitrogen mineralization in paddy soils of the Taihu region of China under anaerobic conditions:Dynamics and model fitting[J]. Acta Pedologica Sinica, 2003, 115(3/4): 161-175. |
[16] |
Pereira, Mouramuniz J, Augustosilva J, et al. Nonlinear models to predict nitrogen mineralization in an oxisol[J]. Scientia Agricola, 2005, 62(4): 395-400. DOI:10.1590/S0103-90162005000400014 |
[17] |
Romano N, Alvarez R, Bono A. Modeling nitrogen mineralization at surface and deep layers of sandy soils[J]. Archives of Agronomy and Soil Science, 2017, 63(6): 870-882. DOI:10.1080/03650340.2016.1241391 |
[18] |
马力, 杨林章, 颜廷梅, 等. 长期施肥水稻土氮素剖面分布及温度对土壤氮素矿化特性的影响[J]. 土壤学报, 2010, 47(2): 286-294. MA Li, YANG Lin-zhang, YAN Ting-mei, et al. Profile distribution and mineraization characteristics of nitrogen in relation to temperature in paddy soil under long-term fertilization[J]. Acta Pedologica Sinica, 2010, 47(2): 286-294. |
[19] |
Moreno-Cornejo J, Zornoza R, Faz A, et al. Effects of pepper crop residues and inorganic fertilizers on soil properties relevant to carbon cycling and broccoli production[J]. Soil Use & Management, 2013, 29(4): 519-530. |
[20] |
张玉玲, 党秀丽, 虞娜, 等. 辽河平原地区长期施肥水稻土氮素矿化及其模拟的研究[J]. 土壤通报, 2008, 39(4): 761-765. ZHANG Yu-ling, DANG Xiu-li, YU Na, et al. Nitrogen mineralization and simulation of paddy soil under different long-term fertilization in Liaohe Plain Region[J]. Chinese Journal of Soil Science, 2008, 39(4): 761-765. |
[21] |
卢红玲, 李世清, 金发会, 等. 黄土高原石灰性土壤长期间隙淋洗淹水培养下的氮素矿化过程及其模拟[J]. 中国农业科学, 2008, 41(10): 3140-3148. LU Hong-ling, LI Shi-qing, JIN Fa-hui, et al. Nitrogen process and simulation of long-term alternate leaching water-logged incubation for calcareous soil on the Loess Plateau[J]. Scientia Agricultura Sinica, 2008, 41(10): 3140-3148. DOI:10.3864/j.issn.0578-1752.2008.10.030 |
[22] |
Gil M V, Carballo M T, Calvo L F. Modelling N mineralization from bovine manure and sewage sludge composts[J]. Bioresource Technology, 2011, 102(2): 863-871. DOI:10.1016/j.biortech.2010.09.010 |
[23] |
Li H L, Han Y, Cai Z C. Modeling nitrogen mineralization in paddy soils of Shanghai Region[J]. Pedosphere, 2003, 13(4): 331-336. |
[24] |
Camargo F A D O, Gianello C, Tedesco M J, et al. Empirical models to predict soil nitrogen mineralization[J]. Ciência Rural, 2002, 32(3): 393-399. DOI:10.1590/S0103-84782002000300005 |
[25] |
刘青丽, 任天志, 李志宏, 等. 植烟黄壤氮素矿化动态模拟研究[J]. 植物营养与肥料学报, 2010, 16(2): 400-406. LIU Qing-li, REN Tian-zhi, LI Zhi-hong, et al. Dynamic simulation of organic nitrogen mineralization in yellow soil of planting tobacco[J]. Plant Nutrition and Fertilizer Science, 2010, 16(2): 400-406. DOI:10.11674/zwyf.2010.0221 |
[26] |
Canali S, Bartolomeo E D, Tittarelli F, et al. Comparison of different laboratory incubation procedures to evaluate nitrogen mineralization in soils amended with aerobic and anaerobic stabilized organic materials[J]. Journal of Food Agriculture & Environment, 2011, 9(2): 540-546. |
[27] |
林俊杰, 张帅, 刘丹, 等. 季节性温度升高对落干期消落带土壤氮矿化影响[J]. 环境科学, 2016, 37(2): 697-702. LIN Jun-jie, ZHANG Shuai, LIU Dan, et al. Effect of seasonal temperature increasing on nitrogen mineralization in soil of the water level fluctuating zone of Three Gorge Tributary during the dry period[J]. Environmental Science, 2016, 37(2): 697-702. |
[28] |
曹竞雄, 韦梦, 陈孟次, 等. 温度对厌氧条件下不同pH水稻土氮素矿化的影响[J]. 中国生态农业学报, 2014, 22(10): 1182-1189. CAO Jing-xiong, WEI Meng, CHEN Meng-ci, et al. Effects of temperature on soil nitrogen mineralization in different pH paddy soils under anaerobic condition[J]. Chinese Journal of Eco-Agriculture, 2014, 22(10): 1182-1189. |
[29] |
王伟, 于兴修, 刘航, 等. 农田土壤氮矿化研究进展[J]. 中国水土保持, 2016(10): 67-71. WANG Wei, YU Xing-xiu, LIU Hang, et al. Advances in nitrogen mineralization in farmland soil[J]. Soil and Water Conservation in China, 2016(10): 67-71. DOI:10.3969/j.issn.1000-0941.2016.10.024 |
[30] |
Sierra J, Marbán L. Nitrogen mineralization pattern of an oxisol of guadeloupe, french west indies[J]. Soil Science Society of America Journal, 2000, 64(6): 2002-2010. DOI:10.2136/sssaj2000.6462002x |
[31] |
王小晓, 黄平, 吴胜军, 等. 土壤氮矿化动力学模型研究进展[J]. 世界科技研究与发展, 2017, 39(2): 164-173. WANG Xiao-xiao, HUANG Ping, WU Sheng-jun, et al. Kinetics modeling of soil nitrogen mineralization:A review[J]. World Sci-Tech R&D, 2017, 39(2): 164-173. |
[32] |
Wu T Y, Ma B L, Liang B C. Quantification of seasonal soil nitrogen mineralization for corn production in eastern Canada[J]. Nutrient Cycling in Agroecosystems, 2008, 81(3): 279-290. DOI:10.1007/s10705-007-9163-x |
[33] |
周涛, 李正魁, 冯露露. 氨氮和硝氮在太湖水华自维持中的不同作用[J]. 中国环境科学, 2013, 33(2): 305-311. ZHOU Tao, LI Zheng-kui, FENG Lu-lu. The different roles of ammonium and nitrate in the bloom self-maintenance of Lake Taihu[J]. China Environmental Science, 2013, 33(2): 305-311. |
[34] |
Shang F Z, Yang P L, Li Y K, et al. Effects of different chemical nitrogenous fertilizer application rates on soil nitrogen leaching and accumulation in deep vadose zone[J]. Transactions of the Chinese Society of Agricultural Engineering, 2012, 28(7): 103-110. |
[35] |
Tang Q, Bao Y H, He X B, et al. Sedimentation and associated trace metal enrichment in the riparian zone of the Three Gorges Reservoir, China[J]. Science of the Total Environment, 2014, 479/480(1): 258-266. |
[36] |
Palanivell P, Ahmed O H, Susilawati K, et al. Mitigating ammonia volatilization from urea in waterlogged condition using clinoptilolite zeolite[J]. International Journal of Agriculture & Biology, 2015, 17(1): 149-155. |
[37] |
Shan L N, He Y F, Chen J, et al. Ammonia volatilization from a Chinese cabbage field under different nitrogen treatments in the Taihu Lake Basin, China[J]. Journal of Environmental Science, 2015, 38(12): 14-23. |
[38] |
曹兵, 贺发云, 徐秋明, 等. 南京郊区番茄地中氮肥的气态氮损失[J]. 土壤学报, 2006, 43(1): 62-68. CAO Bing, HE Fa-yun, XU Qiu-ming, et al. Gaseous losses from fertilizers applied to a tomato field in Nanjing suburbs[J]. Acta Pedologica Sinica, 2006, 43(1): 62-68. |
[39] |
张爱英, 熊高明, 樊大勇, 等. 三峡水库运行对淹没区及消落带植物多样性的影响[J]. 生态学杂志, 2016, 35(9): 2505-2518. ZHANG Ai-ying, XIONG Gao-ming, FAN Da-yong, et al. Effects of damming on plant diversity in the inundated and riparian zones of the Three Gorges Reservoir Area, China[J]. Chinese Journal of Ecology, 2016, 35(9): 2505-2518. |
[40] |
顾春朝, 傅民杰. 不同施肥类型对淹水稻田土壤氮素矿化的影响[J]. 湖北农业科学, 2016, 55(13): 3322-3326. GU Chun-zhao, FU Min-jie. Effects of different fertilizer types on soil nitrogen mineralization in paddy under water-logging condition[J]. Hubei Agricultural Sciences, 2016, 55(13): 3322-3326. |
[41] |
李建兵, 黄冠华. 盐分对粉壤土氮转化的影响[J]. 环境科学研究, 2008, 21(5): 98-103. LI Jian-bing, HUANG Guan-hua. Pilot study of salinity(NaCl) affecting nitrogen transformation in silt loam soil[J]. Research of Environmental Sciences, 2008, 21(5): 98-103. |
[42] |
Yamashita T, Flessa H, John B, et al. Organic matter in density fractions of water-stable aggregates in silty soils:Effect of land use[J]. Soil Biology & Biochemistry, 2006, 38(11): 3222-3234. |
[43] |
于维水, 卢昌艾, 李桂花, 等. 不同施肥制度下中国东部典型土壤易分解与耐分解氮的组分特征[J]. 中国农业科学, 2015, 48(15): 3005-3014. YU Wei-shui, LU Chang-ai, LI Gui-hua, et al. Component characteristics of soil labile and recalcitrant nitrogen under different long-term fertilization systems in East China[J]. Scientia Agricultura Sinica, 2015, 48(15): 3005-3014. DOI:10.3864/j.issn.0578-1752.2015.15.010 |
[44] |
刘杏认, 董云社, 齐玉春, 等. 温带典型草地土壤净氮矿化作用研究[J]. 环境科学, 2007, 28(3): 633-639. LIU Xing-ren, DONG Yun-she, QI Yu-chun, et al. Soil net nitrogen mineralization in the typical temperate grassland[J]. Environmental Science, 2007, 28(3): 633-639. |
[45] |
Harrison-Kirk T, Beare M H, Meenken E D, et al. Soil organic matter and texture affect responses to dry/wet cycles:Changes in soil organic matter fractions and relationships with C and N mineralisation[J]. Soil Biology & Biochemistry, 2014, 74: 50-60. |
[46] |
林俊杰, 刘丹, 张帅, 等. 淹水-落干与季节性温度升高耦合过程对消落带沉积物氮矿化影响[J]. 环境科学, 2017, 38(2): 555-562. LIN Jun-jie, LIU Dan, ZHANG Shuai, et al. Effect of coupling process of wetting-drying cycles and seasonal temperature increasing on sediment nitrogen minerization in the Water Level Fluctuating Zone[J]. Environmental Science, 2017, 38(2): 555-562. |
[47] |
Haer H S, Benbi D K. Modeling nitrogen mineralization kinetics in arable soils of semiarid india[J]. Arid Land Research & Management, 2003, 17(2): 153-168. |
[48] |
Schomberg H H, Wietholter S, Griffin T S, et al. Assessing indices for predicting potential nitrogen mineralization in soils under different management systems[J]. Soil Science Society of America Journal, 2009, 73(5): 1575-1586. DOI:10.2136/sssaj2008.0303 |
[49] |
周吉利, 邹刚华, 彭佩钦, 等. 中亚热带典型红壤区土壤氮矿化动力学参数估算[J]. 农业现代化研究, 2015, 36(4): 702-707. ZHOU Ji-li, ZOU Gang-hua, PENG Pei-qin, et al. Estimating the kinetic parameters of soil organic nitrogen mineralization for various land use types in a typical hilly red-soil region in subtropical central China[J]. Research of Agricultural Modernization, 2015, 36(4): 702-707. |