文章信息
- 魏天娇, 管冬兴, 方文, 罗军
- WEI Tian-jiao, GUAN Dong-xing, FANG Wen, LUO Jun
- 梯度扩散薄膜技术(DGT)的理论及其在环境中的应用Ⅲ——植物有效性评价的理论基础与应用潜力
- Theory and application of diffusive gradients in thin-films(DGT) in the environment Ⅲ: Theoretical basis and application potential in phytoavailability assessment
- 农业环境科学学报, 2018, 37(5): 841-849
- Journal of Agro-Environment Science, 2018, 37(5): 841-849
- http://dx.doi.org/10.11654/jaes.2018-0341
文章历史
- 收稿日期: 2018-03-14
- 修回日期: 2018-04-16
土壤、自然水体、沉积物等环境介质中的磷和金属(类金属)浓度处于适宜范围是实现生态系统正常功能和良好农业产出的重要前提。环境中磷过量可导致富营养化现象,而金属(类金属)过量可对植物造成毒害。对于特定环境介质中的磷和金属(类金属),具有生物有效性的部分才能被植物吸收利用并产生环境影响。因此,解释和预测这些元素的生物有效性成了近些年的研究热点。生物有效性目前没有明确一致的定义[1-2],但一些研究者给出了生物有效性的概念,这些概念总体上可分为基于化学的和基于生物学的两个方面[3]。McCarthy和Mackay[4]指出,生物有效性评价需综合考虑物理化学作用驱动的供给和生物学驱动的吸收这两个阶段的动态过程。因此,不同概念的实质都在于,将生物体与环境中的物理化学过程综合起来考虑其潜在的相互关系。正因如此,植物特定的生理特性以及环境中的地球化学条件均是影响磷和金属(类金属)等生物有效性的重要因素。综合考虑多过程、多机制的耦合作用是现有生物有效性评价方法的重要发展方向。
梯度扩散薄膜技术(DGT)是一种原位监测和形态分析技术[5]。自1999年以来[6],诸多研究将DGT广泛应用于土壤、水体、沉积物等多种环境介质中磷和金属(类金属)的植物有效性解释和预测。大量研究发现,DGT技术预测与其他传统的植物有效性评价技术相比,能更好地反映植物对磷和金属(类金属)的吸收[7]。与基于分配平衡原理的有效性评估方法(如毒性浸出实验等)不同,DGT技术是一种基于解离、扩散动力学的方法。DGT技术测定的有效态浓度包括溶液中的离子态物质、络合态物质中的可解离部分以及固相向液相的补给部分,该浓度反映了环境介质对目标元素的再补给能力以及对补给过程有所贡献的形态[8]。植物对磷和金属(类金属)的吸收消耗过程也引起了目标物质从环境介质到植物体表面的动力学过程,这与DGT吸收待测物质的过程机理类似,因此DGT技术是一种研究植物有效性机制的工具。
在此,本文主要关注DGT技术对磷和金属(类金属)的植物有效性评价,简要阐述了DGT技术基于动力学过程进行有效性测定的原理;剖析DGT技术涉及的动力学过程与植物吸收机制的相似性与差异性;详细评估DGT技术在预测植物吸收磷和金属(类金属)的效果;并基于以上内容,对DGT技术在植物有效性方面的应用进行总结和展望。
1 DGT技术基本原理DGT是基于Fick第一扩散定律开发的污染物原位研究技术,其由最内侧的吸附层、覆盖于其上的特定厚度扩散层、起保护作用的滤膜组成[9-11]。吸附膜中的吸附材料与特定的磷和金属(类金属)有很强的结合能力,溶液中的待测物透过扩散膜到达吸附膜后立即被吸附而消耗,在扩散层-吸附层界面上浓度降为零,从而形成了贯穿整个扩散层的浓度梯度[8]。这一浓度梯度驱动着待测物以一定通量从扩散层外侧的环境介质向扩散层内侧的吸附层不断扩散。基于Fick第一定律,则待测物的扩散通量为:
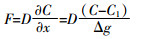
式中:D为待测物质在扩散膜中的扩散系数;C为待测物质在溶液中的浓度;C1为待测物质在吸附胶与扩散胶界面处的浓度;Δg为扩散胶的厚度。在吸附胶饱和之前,C1等于0。因此,式(1)可以转化为式(2):

通量F也可以表述为:

式中:M为待测物质在吸附胶中的积累量;A为DGT装置窗口的面积;t为吸附时间。将式(3)带入式(2)得到:

DGT在测定无机物时,通常认为只有自由离子能被吸附层吸附[12-13]。当环境介质中的自由离子持续迁移并积累到吸附层上,就需要从动力学角度考虑环境介质中其他络合物对自由离子的补充[14]。当自由离子被DGT吸附引起靠近DGT装置区域的浓度下降,环境介质溶液中原有的平衡被打破时,与有机物等配体络合的相应元素就会发生解离,以维持溶液中原有的自由离子浓度。同时,动态交换过程也发生在溶液和固相之间[15]。这两种方式供给自由离子的能力取决于待测物解离或解吸的速率。当配合物解离或固相解吸释放自由离子的速率足够快时,被固相吸附的相应元素就会对溶液起到很强的缓冲作用,自由离子浓度的降低也就非常少,溶液保持了原有的平衡状态。如果自由离子释放的速率很慢,DGT界面附近的自由离子浓度逐渐降低,则会改变系统的平衡状态,使得系统中的化学平衡向解离或解吸出自由离子的方向移动[16]。图 1以土壤为例说明了这一过程。最终,环境介质中的自由离子以及能够快速从固相和络合态解离的形态,即为DGT测量得到的有效态。
理解植物吸收磷以及金属(类金属)离子的相关机制和平衡模型(Equilibrium models),是对比DGT测量得到的有效态浓度与植物吸收的浓度的前提。相比于溶液中的溶质以扩散形式供应的速率,如果植物吸收自由离子的速率非常缓慢,那么吸收过程对该区域内元素形态分布的扰动就微乎其微,生物膜表面自由离子的浓度也不会降低,整个体系的平衡未被打破。这种情况下限速的是生物吸收的速率,该情况通常被称为自由离子活度模型(FIAM),吸收量与自由离子活度成正比[18]。这个模型适用于许多浮游植物的吸收过程。生物配体模型(BLM)在FIAM的基础上考虑了竞争离子的影响,该模型认为,待吸收的离子需首先结合在生物膜表面的结合位点,进而被植物体吸收,而环境介质中Ca2+等其他离子会与目标离子竞争这些结合位点,导致植物体对目标离子的吸收通量下降。如果环境中存在能够解离出目标离子的络合物,则这些络合物解离出的离子也对扩散通量有贡献,该离子总的扩散通量将会提高。图 2a以示意图的形式描述了这一模型。BLM成功预测了许多水生生物对金属的吸收[19-20]。总的来说,FIAM和BLM适用的情况仅限于体系中供给的自由离子流量大于生物吸收的流量,即溶液所受的扰动可被忽略的情况。
![]() |
a.显示了内化通量与扩散随自由离子浓度的变化关系,以及它们如何受可解离的络合物(Labile complex)存在(扩散通量)或吸收竞争离子存在(内化通量)的影响;b.显示了植物吸收通量和自由离子浓度的理论关系 a. Schematic diagram showing the internalization flux and diffusion flux as a function of free ion concentration and how they are affected by the presence of labile complexes(diffusion flux)or the presence of ions that compete for uptake(internalization flux). b. Theoretical relationships between uptake fluxes by a plant and free ion concentration 图 2 植物吸收机制示意图(改编自Davison[16]和Degryse等[22]) Figure 2 Schematic diagram of plant uptake mechanisms(Adopted from Davison[16] and Degryse et al[22]) |
如果生物吸收速率非常快,溶质扩散的速率相对很慢,生物膜表面自由离子的浓度就会降低,进而溶液平衡被打破,此时溶质扩散的速率是植物吸收待测物的限速步骤。这种情况下,生物膜表面自由离子的浓度几乎为零,因此形成了从溶液主体到生物膜表面的浓度梯度,这与DGT技术的原理非常相似[16]。在形成浓度梯度的区域,自由离子浓度低于主体溶液,配合物发生解离,其产生的自由离子也对供应植物吸收的流量有所贡献。不同植物平衡模型下,系统中自由离子浓度与通过生物膜的待测物通量的关系如图 2b所示,简要来说,如果环境介质通过扩散提供的自由离子通量高于植物的吸收能力,那么植物吸收的限制因素为植物自身,如果植物自身所能达到的最大吸收通量高于环境介质能提供的最高的自由离子扩散通量,那么扩散通量则成为吸收过程的限制因素。上述讨论表明,被化学方法测定的有效态不一定全部被植物吸收,而是包括了潜在的植物有效性部分,仅当生物吸收速率非常快时有效态才能全部被吸收[21]。
2.2 扩散层对DGT模拟植物吸收的影响DGT有效态不是指某些固定的形态,除了自由离子,还包括在一定时间内能够解离出自由离子的形态。这个时间范围主要是由DGT扩散层厚度决定的。只有在络合物穿过扩散膜到达吸附膜的这段时间内能够解离的部分才对DGT所测的通量有贡献。同样地,在植物吸收过程受扩散限制的情况下,只有在络合物穿过生物膜外侧的浓度梯度区域到达生物膜表面的这段时间内,解离出的自由离子形态,才能被植物吸收,即对扩散通量有贡献。在扩散控制的条件下,相似的扩散层厚度意味着相近的扩散时间。扩散层厚度相近可以更准确地反映扩散控制条件下植物吸收元素的真实情况。van Leeuwen等[21]发现,相比于比其他动力学技术,DGT的扩散层与植物扩散层的相似程度尤其高。需要注意的是,虽然某些情况下DGT扩散通量与植物吸收通量相似,但二者也非完全吻合,主要因为:(1)不同的土壤水分含量;(2)CDGT一定程度上受放置时间的影响;(3)DGT与根系几何形状不同;(4)植物诱导的根际变化[22]。
3 DGT在植物吸收机制研究方面的应用FIAM或BLM模型适用于模拟水体中植物体对磷及金属元素的缓慢吸收,而DGT作为基于扩散的动力学技术,适用于模拟植物体吸收速率较快、扩散补给是限速过程的情况。DGT测得的有效态浓度与植物吸收的实际浓度之间的关系是推导植物对磷和金属吸收机制的关键证据。
Degryse等[23-24]将DGT用于陆生植物水培实验,探究菠菜对Cd的吸收机制。水培实验中,向Cd溶液加入不同浓度的合成配体,同时保持自由离子浓度和总浓度不变,测定菠菜对Cd的吸收。结果显示菠菜对Cd的吸收量随离解速率增加而上升,并与DGT测定结果相关性良好,证明这一体系下菠菜对Cd的吸收受扩散作用限制,此时FIAM等模型不适用于模拟植物的吸收过程。对油菜籽的实验也得到了类似的结果。Oporto等[25]对比了在不同浓度Cd溶液下DGT对菠菜吸收Cd能力的预测情况。低浓度(1 nmol·L-1)Cd2+条件下,氯配合物的存在增加了菠菜吸收的通量,但高浓度(1 μmol·L-1)Cd2+条件下菠菜对Cd的吸收未受氯配合物的影响。DGT可以较好地模拟低浓度Cd2+条件下菠菜对Cd的吸收。这证明了在低浓度的Cd2+条件下植物对Cd的吸收过程主要受扩散限制,而高浓度Cd2+条件下植物的吸收速率已经达到饱和,对Cd的吸收主要受自身吸收速率的限制。将DGT应用于番茄和菠菜对Cd和Ni吸收的实验发现,DGT可解离的配合物(Labile complex)的存在增大了植物对Cd的吸收,但对Ni没有影响。证实在环境相关浓度下,番茄和菠菜对Cd吸收受扩散控制,而Ni则相反[24]。Wang等[26]研究小麦对Zn的吸收机制采用了微分脉冲阳极溶出伏安法(DPASV)和DGT两种动力学方法,发现二者与植物吸收均有很好的相关性,验证了扩散作用对小麦吸收Zn的控制作用。
Luo等[17]在土培条件下研究了超富集植物和非超富集植物对Cd和Ni的吸收机制,探究植物中Cd和Ni的含量与DGT测定的有效态浓度的相关性。研究结果显示,超富集植物对Ni的吸收受扩散过程控制,非超富集植物对Ni的吸收受植物吸收能力的控制,而两种植物对Cd的吸收均受扩散过程的控制。Luo等[2]进一步研究小萝卜对13种土壤中Ni的吸收与3种方法(DGT、土壤溶液、Ni2+)的相关性。研究发现低浓度下只有DGT与小萝卜体内Ni浓度有较好的相关性,说明此时Ni的吸收受扩散控制,而高浓度下这3种方法均与小萝卜体内Ni浓度相关性较高,此结果表明当植物吸收量与DGT有效态浓度相关性较好时,不能完全证明植物对目标物质的吸收受扩散过程控制,可能是因为二者恰好具有相似的吸收通量。
4 DGT在评价陆生植物有效性方面的应用当DGT技术应用于土壤中有效态浓度的分析,其综合考虑了土壤溶液中目标物质的交换与扩散过程,以及目标物质从土壤固相向液相补给的动态平衡[2, 27]。DGT装置放置于土壤中后,临近DGT暴露窗口区域的土壤溶液中目标物质浓度开始降低,体系中原有的平衡状态受到了扰动。此时,土壤固相中可交换态的目标物质释放出来,向土壤溶液补给,同时,土壤溶液中的配合物也发生解离,这两个过程产生的自由离子均会对DGT的吸收通量做出贡献。已有文献[22, 28]从模型角度对植物吸收量和DGT测定的有效态浓度相似之处进行讨论。简言之,有两种典型情况:(1)土壤溶液中目标物质的浓度很低,植物需求高,在界面上目标物质浓度不断下降,此时DGT测定的有效态浓度与植物吸收量存在较好的相关性,该情况一般发生于土壤中微量元素含量不足时;(2)植物需求低,土壤溶液中目标物质浓度高,界面上目标物质浓度变化很小,此时DGT测定的有效态浓度可能与植物吸收量的相关性较差,该情况一般发生于土壤污染程度较高并对植物产生毒害作用时[29]。
在土壤环境中利用DGT研究生物有效性时,DGT测得的浓度数据有两种表达方式,即CDGT和CE。如前所述,CDGT反映了一段时间内DGT-土壤界面上的平均浓度,包括瞬时界面浓度下降后通过土壤固相解吸出的离子。土壤固相对土壤溶液活性物质的补充能力受多方面因素的影响,比如目标物质自身特性(吸附能力和扩散能力)和土壤性质(土壤曲折度、土壤密度)等。因此,土壤固相对活性物质的补充能力存在两种极端情况:完全持续型和单扩散型[30]。完全持续型指土壤固相补充待测物质的速率足够快且不会衰竭,此时DGT-土壤界面上的浓度则不会下降,CDGT与土壤溶液初始的瞬时浓度非常接近。单扩散型指土壤固相对土壤溶液待测物质的补充能力非常弱,当DGT-土壤界面上的待测物质被移除后,只能靠远离该界面的高浓度土壤溶液以扩散的方式补充到DGT-土壤界面。介于两种之间的被称为部分持续型。为了考察土壤固相和土壤溶液中全部能被有效利用的待测物质浓度,DGT用于研究土壤环境时常使用有效浓度CE这一概念[31]。

式中:Rdiff指土壤中只存在溶液扩散补充而没有固相补充的情况下,DGT-土壤理论界面浓度与土壤溶液浓度的比值。Rdiff取决于土壤性质,可以通过2D DIFS模型(2D DGT induced fluxes in soils)计算得到。通过Rdiff对CDGT进行校正,得到土壤中全部能被有效利用的待测物质浓度。一般情况下,CE和CDGT有很强的相关性。
DGT以及其他方法对磷和金属植物有效性的评估效果已被广泛探讨(表 1)。大多数研究发现DGT测定的有效态浓度与植物体内的金属和磷浓度有较好的相关性,但也有一些例外。Nawara等[32]用包括DGT在内的5种方法预测了十余种长期田间试验土壤中磷的生物有效性,并将这些方法分为预测土壤有效态磷的总量(Q)和强度(Ⅰ)两类,发现以DGT为代表的Ⅰ方法与Q方法相比没有明显优势。Dai等[33]比较了DGT、化学提取法和总量法预测Cd植物有效性的效果,发现虽然DGT与植物体内Cd含量相关性略高于其他方法,但各方法预测效果均受土壤性质的影响。Zhang等[34]发现DGT有效态金属(Cd、Cu、Ni、Pb、Zn)与小白菜体内金属含量并未取得很好的相关性,同时,该研究将金属吸收过程分为吸附在根表、根表转移至根内、根部转运至地上部分等几个步骤进行预测,得到了很好的结果,由此可见金属吸收过程受复杂的生物因素影响,而DGT无法反映生物因素对植物有效性的影响。根据DGT技术预测植物吸收元素的案例对比,可以发现当土壤过程作为限制因素影响植物吸收的时候,DGT技术相对其他方法能够比较好地反映植物吸收,这也说明DGT技术比其他化学方法能更好地研究磷和金属的土壤动力学过程,并反映其对植物有效性的影响。
此外,DGT技术作为一种研究溶质一维或二维空间分布的工具,能够构建高度异质性的土壤-根系界面的高分辨率(≤mm)剖面图,可以显示出土壤亚毫米结构范围内的地球化学反应[35]。通过联用平面光极等二维高分辨技术获取环境参数的二维分布信息,可以进一步解释磷和金属(类金属)与植物相互作用的机制[36]。
5 DGT在评价水生植物有效性方面的应用目前,水体环境中磷和金属(类金属)的生物有效性评价多采用BLM和FIAM模型,用DGT评价生物有效性的研究远少于土壤,利用DGT对水生植物有效性进行评价的结果也不甚一致。DGT和中空纤维渗透液膜(HFPLM)(自由离子)预测黑海绿藻(Chlorella salina)对Cd、Cu、Ni和Pb的吸收均取得了较好的结果[37]。Schintu等[38]研究了地中海沿岸5个采样点的藻类对金属的吸收,发现只有Pb对Padina pavonica L.的生物有效性与DGT测定值有显著相关。水生苔藓(Fontinalis antipyretica)的中宇宙研究[39]发现DGT对苔藓吸收Cu的预测比Cu2+选择电极好,这可能反映了与自由离子活性相比,将DGT与阳离子竞争模型相结合是预测水生植物吸收磷和金属的相对较好的方法。然而在野外实验中,DGT与水生苔藓中多种金属浓度并无显著相关性,这可能是因为野外生长的苔藓中含有特定的颗粒状金属,无法透过扩散层而被DGT测到。
在沉积物环境中,DGT多被用于对动物体(双壳类、螺类等)生物有效性的评估[40-42],实验室和野外应用的对比研究取得了较好的效果。Ren等[43]探讨了DGT与BCR提取法的关系,认为DGT具有评价沉积物中植物有效性的潜力。Song等[44]利用DGT研究了河流系统的沉积物上生长的芦苇(Phragmites australis)对多种金属和类金属的生物累积,发现DGT测得的沉积物中有效态浓度与芦苇体内累积的Cr、Zn、Cu、Cd有很强的相关性,而与As相关性很弱。利用DGT对水生植物Zizania latifolia和Myriophyllum verticiilaturn体内和沉积物中的P、Cu、Cd、Pb、Zn的研究表明,DGT能够提供沉积物中植物吸收磷和金属(类金属)的原位、动力学信息,从动力学角度证明DGT能够预测沉积物中水生植物有效性[45-46]。
此外,已有研究初步发现了DGT在污水处理厂脱水污泥[70]等环境介质中评价植物有效性的潜力。
6 展望由于DGT技术有基于动力学的元素形态分析能力,其在测量元素的植物生物有效性方面有独特的优势,能更好地研究磷和金属(类金属)的植物有效性。通过探讨植物吸收过程引起的元素形态变化的动力学过程,DGT可以作为深入揭示植物吸收磷和金属(类金属)等物质的化学和生物学机理的工具之一。通过结合生态毒理学研究手段和模型,可以进一步建立和推广以DGT为基础的普适的土壤环境质量评价体系。
[1] |
Nolan A L, Lombi E, McLaughlin M J. Metal bioaccumulation and toxicity in soils:Why bother with speciation?[J]. Australian Journal of Chemistry, 2003, 56(2/3): 77-91. |
[2] |
Luo J, Cheng H, Ren J, et al. Mechanistic insights from DGT and soil solution measurements on the uptake of Ni and Cd by radish[J]. Environmental Science & Technology, 2014, 48(13): 7305-7313. |
[3] |
Barber S A. Soil nutrient bioavailability: A mechanistic approach[M]. New York: John Wiley & Sons, 1995.
|
[4] |
McCarty L S, Mackay D. Enhancing ecotoxicological modeling and assessment. Body residues and modes of toxic action[J]. Environmental Science and Technology, 1993, 27(9): 1719-1728. |
[5] |
Davison W, Zhang H. In situ speciation measurements of trace components in natural-waters using thin-film gels[J]. Nature, 1994, 367(6463): 546-548. DOI:10.1038/367546a0 |
[6] |
Davison W, Hooda P S, Zhang H, et al. DGT measured fluxes as surrogates for uptake of metals by plants[J]. Advances in Environmental Research, 2000, 3(4): 550-555. |
[7] |
Zhang H, Davison W. Use of diffusive gradients in thin-films for studies of chemical speciation and bioavailability[J]. Environmental Chemistry, 2015, 12(2): 85-101. |
[8] |
Davison W, Zhang H. Progress in understanding the use of diffusive gradients in thin films(DGT):Back to basics[J]. Environmental Chemistry, 2012, 9(1): 1-13. |
[9] |
Zhang H, Davison W. Performance characteristics of diffusion gradients in thin films for the in situ measurement of trace metals in aqueous solution[J]. Analytical Chemistry, 1995, 67(19): 3391-3400. DOI:10.1021/ac00115a005 |
[10] |
Guan D X, Williams P N, Luo J, et al. Novel precipitated zirconia-based DGT technique for high-resolution imaging of oxyanions in waters and sediments[J]. Environmental Science & Technology, 2015, 49(6): 3653-3661. |
[11] |
周春羊, 管冬兴, 韩永和, 等. 基于梯度扩散薄膜技术的太湖金属和营养盐区域污染特征分析[J]. 农业环境科学学报, 2016, 35(6): 1144-1152. ZHOU Chun-yang, GUAN Dong-xing, HAN Yong-he, et al. Pollution characteristics of metals and nutrients in different regions of Lake Taihu based on diffusive gradients in thin-films[J]. Journal of Agro-Environment Science, 2016, 35(6): 1144-1152. DOI:10.11654/jaes.2016.06.017 |
[12] |
Zhang H, Davison W, Gadi R, et al. In situ measurement of dissolved phosphorus in natural waters using DGT[J]. Analytica Chimica Acta, 1998, 370(1): 29-38. DOI:10.1016/S0003-2670(98)00250-5 |
[13] |
Zhang H, Davison W, Knight B, et al. In situ measurements of solution concentrations and fluxes of trace metals in soils using DGT[J]. Environmental Science & Technology, 1998, 32(5): 704-710. |
[14] |
Zhao C M, Campbell P G C, Wilkinson K J. When are metal complexes bioavailable?[J]. Environmental Chemistry, 2016, 13(3): 425-433. |
[15] |
Guan D X, Zheng J L, Luo J, et al. A diffusive gradients in thin-films technique for the assessment of bisphenols desorption from soils[J]. Journal of Hazardous Materials, 2017, 331: 321-328. DOI:10.1016/j.jhazmat.2017.02.053 |
[16] |
Davison W. Diffusive gradients in thin-films for environmental measurements[M]. Cambridge: Cambridge University Press, 2016.
|
[17] |
Luo J, Zhang H, Zhao F J, et al. Distinguishing diffusional and plant control of Cd and Ni uptake by hyperaccumulator and nonhyperaccumulator plants[J]. Environmental Science & Technology, 2010, 44(17): 6636-6641. |
[18] |
Campbell P G C. Interactions between trace metals and aquatic organisms:A critique of the free-ion activity model[J]. Metal Speciation and Bioavailability, 1994, 45-102. |
[19] |
Di Toro D M, Allen H E, Bergman H L, et al. Biotic ligand model of the acute toxicity of metals. 1. Technical basis[J]. Environmental Toxicology and Chemistry, 2001, 20(10): 2383-2396. DOI:10.1002/etc.v20:10 |
[20] |
Ardestani M M, van Straalen N M, van Gestel C A. The relationship between metal toxicity and biotic ligand binding affinities in aquatic and soil organisms:A review[J]. Environmental Pollution, 2014, 195: 133-147. DOI:10.1016/j.envpol.2014.08.020 |
[21] |
van Leeuwen H P, Town R M, Buffle J, et al. Dynamic speciation analysis and bioavailability of metals in aquatic systems[J]. Environmental Science & Technology, 2005, 39(22): 8545-8556. |
[22] |
Degryse F, Smolders E, Zhang H, et al. Predicting availability of mineral elements to plants with the DGT technique:A review of experimental data and interpretation by modelling[J]. Environmental Chemistry, 2009, 6(3): 198-218. DOI:10.1071/EN09010 |
[23] |
Degryse F, Smolders E, Merckx R. Labile Cd complexes increase Cd availability to plants[J]. Environmental Science & Technology, 2006, 40(3): 830-836. |
[24] |
Degryse F, Smolders E. Cadmium and nickel uptake by tomato and spinach seedlings:Plant or transport control?[J]. Environmental Chemistry, 2012, 9(1): 48-54. DOI:10.1071/EN11060 |
[25] |
Oporto C, Smolders E, Degryse F, et al. DGT-measured fluxes explain the chloride-enhanced cadmium uptake by plants at low but not at high Cd supply[J]. Plant and Soil, 2009, 318(1): 127-135. |
[26] |
Wang P, Zhou D M, Luo X S, et al. Effects of Zn-complexes on zinc uptake by wheat(Triticum aestivum) roots:A comprehensive consideration of physical, chemical and biological processes on biouptake[J]. Plant and Soil, 2009, 316(1/2): 177-192. |
[27] |
Guan D X, Williams P N, Xu H C, et al. High-resolution measurement and mapping of tungstate in waters, soils and sediments using the low-disturbance DGT sampling technique[J]. Journal of Hazardous Materials, 2016, 316: 69-76. DOI:10.1016/j.jhazmat.2016.05.026 |
[28] |
Lehto N J, Davison W, Zhang H, et al. Theoretical comparison of how soil processes affect uptake of metals by diffusive gradients in thinfilms and plants[J]. Journal of Environmental Quality, 2006, 35(5): 1903-1913. DOI:10.2134/jeq2005.0422 |
[29] |
Lehto N J, Davison W, Zhang H, et al. Analysis of micro-nutrient behaviour in the rhizosphere using a DGT parameterised dynamic plant uptake model[J]. Plant and Soil, 2006, 282(1/2): 227-238. |
[30] |
罗军, 王晓蓉, 张昊, 等. 梯度扩散薄膜技术(DGT)的理论及其在环境中的应用Ⅰ:工作原理、特性与在土壤中的应用[J]. 农业环境科学学报, 2011, 30(2): 205-213. LUO Jun, WANG Xiao-rong, ZHANG Hao, et al. Theory and application of diffusive gradients in thin-films in soils[J]. Journal of Agro-Environment Science, 2011, 30(2): 205-213. |
[31] |
Zhang H, Zhao F J, Sun B, et al. A new method to measure effective soil solution concentration predicts copper availability to plants[J]. Environmental Science & Technology, 2001, 35(12): 2602-2607. |
[32] |
Nawara S, Van Dael T, Merckx R, et al. A comparison of soil tests for available phosphorus in long-term field experiments in Europe[J]. European Journal of Soil Science, 2017, 68(6): 873-885. DOI:10.1111/ejss.12486 |
[33] |
Dai Y, Nasir M, Zhang Y, et al. Comparison of DGT with traditional methods for assessing cadmium bioavailability to Brassica chinensis in different soils[J]. Scientific Reports, 2017, 7(1): 14206. DOI:10.1038/s41598-017-13820-3 |
[34] |
Zhang S, Song J, Gao H, et al. Improving prediction of metal uptake by Chinese cabbage(Brassica pekinensis L.) based on a soil-plant stepwise analysis[J]. Science of the Total Environment, 2016, 569/570: 1595-1605. DOI:10.1016/j.scitotenv.2016.07.007 |
[35] |
Cai C, Williams P N, Li H, et al. Development and application of the diffusive gradients in thin films technique for the measurement of nitrate in soils[J]. Analytical Chemistry, 2017, 89(2): 1178-1184. DOI:10.1021/acs.analchem.6b03609 |
[36] |
房煦, 罗军, 高悦, 等. 梯度扩散薄膜技术(DGT)的理论及其在环境中的应用Ⅱ:土壤与沉积物原位高分辨分析中的方法与应用[J]. 农业环境科学学报, 2017, 36(9): 1693-1702. FANG Xu, LUO Jun, GAO Yue, et al. Theory and application of diffusive gradients in thin-films in the environment:High-resolution analysis and its applications in soils and sediments[J]. Journal of Agro-Environment Science, 2017, 36(9): 1693-1702. DOI:10.11654/jaes.2017-0454 |
[37] |
Slaveykova V I, Karadjova I B, Karadjov M, et al. Trace metal speciation and bioavailability in surface waters of the black sea coastal area evaluated by HF-PLM and DGT[J]. Environmental Science & Technology, 2009, 43(6): 1798-1803. |
[38] |
Schintu M, Marras B, Durante L, et al. Macroalgae and DGT as indicators of available trace metals in marine coastal waters near a lead zinc smelter[J]. Environmental Monitoring and Assessment, 2010, 167(1): 653-661. |
[39] |
Vannuci-Silva M, de Souza J M, de Oliveira F F, et al. Bioavailability of metals at a southeastern brazilian coastal area of high environmental concern under anthropic influence:Evaluation using transplanted bivalves(Nodipecten nodosus) and the DGT technique[J]. Water, Air, and Soil Pollution, 2017, 228(6): 222. DOI:10.1007/s11270-017-3387-4 |
[40] |
Lydy M J, Landrum P F, Oen A M P, et al. Passive sampling methods for contaminated sediments:State of the science for organic contaminants[J]. Integrated Environmental Assessment and Management, 2014, 10(2): 167-178. DOI:10.1002/ieam.1503 |
[41] |
Amirbahman A, Massey D I, Lotufo G, et al. Assessment of mercury bioavailability to benthic macroinvertebrates using diffusive gradients in thin films(DGT)[J]. Environmental Science-Processes & Impacts, 2013, 15(11): 2104-2114. |
[42] |
Ren J H, Luo J, Ma H R, et al. Bioavailability and oxidative stress of cadmium to Corbicula fluminea[J]. Environ Sci Process Impacts, 2013, 15(4): 860-869. DOI:10.1039/c3em30288a |
[43] |
Ren J, Williams P N, Luo J, et al. Sediment metal bioavailability in Lake Taihu, China:Evaluation of sequential extraction, DGT, and PBET techniques[J]. Environmental Science and Pollution Research, 2015, 22(17): 12919-12928. DOI:10.1007/s11356-015-4565-9 |
[44] |
Song Z X, Shan B Q, Tang W Z. Evaluating the diffusive gradients in thin films technique for the prediction of metal bioaccumulation in plants grown in river sediments[J]. Journal of Hazardous Materials, 2018, 344: 360-368. DOI:10.1016/j.jhazmat.2017.10.049 |
[45] |
Wu Z H, Wang S R, Luo J. Transfer kinetics of phosphorus(P) in macrophyte rhizosphere and phytoremoval performance for lake sediments using DGT technique[J]. Journal of Hazardous Materials, 2018, 350: 189-200. DOI:10.1016/j.jhazmat.2018.02.005 |
[46] |
Wang S R, Wu Z H, Luo J. Transfer mechanism, uptake kinetic process, and bioavailability of P, Cu, Cd, Pb, and Zn in macrophyte rhizosphere using diffusive gradients in thin films[J]. Environmental Science & Technology, 2018, 52(3): 1096-1108. |
[47] |
Williams P N, Zhang H, Davison W, et al. Organic matter-solid phase interactions are critical for predicting arsenic release and plant uptake in Bangladesh paddy soils[J]. Environmental Science & Technology, 2011, 45(14): 6080-6087. |
[48] |
Ngo L K, Pinch B M, Bennett W W, et al. Assessing the uptake of arsenic and antimony from contaminated soil by radish(Raphanus sativus) using DGT and selective extractions[J]. Environmental Pollution, 2016, 216: 104-114. DOI:10.1016/j.envpol.2016.05.027 |
[49] |
Williams P N, Zhang H, Davison W, et al. Evaluation of in situ DGT measurements for predicting the concentration of Cd in Chinese field-cultivated rice:Impact of soil Cd:Zn ratios[J]. Environmental Science & Technology, 2012, 46(15): 8009-8016. |
[50] |
Perez A L, Anderson K A. DGT estimates cadmium accumulation in wheat and potato from phosphate fertilizer applications[J]. Science of the Total Environment, 2009, 407(18): 5096-5103. DOI:10.1016/j.scitotenv.2009.05.045 |
[51] |
Song J, Zhao F J, Luo Y M, et al. Copper uptake by Elsholtzia splendens and Silene vulgaris and assessment of copper phytoavailability in contaminated soils[J]. Environmental Pollution, 2004, 128(3): 307-315. DOI:10.1016/j.envpol.2003.09.019 |
[52] |
Bravin M N, Michaud A M, Larabi B, et al. Rhizotest:A plant-based biotest to account for rhizosphere processes when assessing copper bioavailability[J]. Environmental Pollution, 2010, 158(10): 3330-3337. DOI:10.1016/j.envpol.2010.07.029 |
[53] |
Zhang H, Lombi E, Smolders E, et al. Kinetics of Zn release in soils and prediction of Zn concentration in plants using diffusive gradients in thin films[J]. Environmental Science & Technology, 2004, 38(13): 3608-3613. |
[54] |
Koster M, Reijnders L, van Oost N R, et al. Comparison of the method of diffusive gels in thin films with conventional extraction techniques for evaluating zinc accumulation in plants and isopods[J]. Environmental Pollution, 2005, 133(1): 103-116. DOI:10.1016/j.envpol.2004.05.022 |
[55] |
Nolan A L, Zhang H, McLaughlin M J. Prediction of zinc, cadmium, lead, and copper availability to wheat in contaminated soils using chemical speciation, diffusive gradients in thin films, extraction, and isotopic dilution techniques[J]. Journal of Environmental Quality, 2005, 34(2): 496-507. DOI:10.2134/jeq2005.0496 |
[56] |
Chapman E E V, Dave G, Murimboh J D. Bioavailability as a factor in risk assessment of metal-contaminated soil[J]. Water, Air, and Soil Pollution, 2012, 223(6): 2907-2922. DOI:10.1007/s11270-012-1074-z |
[57] |
Agbenin J O, Welp G. Bioavailability of copper, cadmium, zinc, and lead in tropical savanna soils assessed by diffusive gradient in thin films(DGT) and ion exchange resin membranes[J]. Environmental Monitoring and Assessment, 2012, 184(4): 2275-84. DOI:10.1007/s10661-011-2116-5 |
[58] |
Tian Y, Wang X, Luo J, et al. Evaluation of holistic approaches to predicting the concentrations of metals in field-cultivated rice[J]. Environmental Science & Technology, 2008, 42(20): 7649-7654. |
[59] |
Soriano-Disla J M, Speir T W, Gómez I, et al. Evaluation of different extraction methods for the assessment of heavy metal bioavailability in various soils[J]. Water, Air, and Soil Pollution, 2010, 213(1): 471-483. |
[60] |
Cornu J Y, Denaix L. Prediction of zinc and cadmium phytoavailability within a contaminated agricultural site using DGT[J]. Environmental Chemistry, 2006, 3(1): 61-64. |
[61] |
Muhammad I, Puschenreiter M, Wenzel W W. Cadmium and Zn availability as affected by pH manipulation and its assessment by soil extraction, DGT and indicator plants[J]. Science of the Total Environment, 2012, 416: 490-500. DOI:10.1016/j.scitotenv.2011.11.029 |
[62] |
Nowack B, Koehler S, Schulin R. Use of diffusive gradients in thin films(DGT) in undisturbed field soils[J]. Environmental Science & Technology, 2004, 38(4): 1133-1138. |
[63] |
Ahumada I, Ascar L, Pedraza C, et al. Determination of the bioavailable fraction of Cu and Zn in soils amended with biosolids as determined by diffusive gradients in thin films(DGT), BCR sequential extraction, and ryegrass plant[J]. Water, Air, and Soil Pollution, 2011, 219(1/2/3/4): 225-237. |
[64] |
Puschenreiter M, Wittstock F, Friesl-Hanl W, et al. Predictability of the Zn and Cd phytoextraction efficiency of a Salix smithiana clone by DGT and conventional bioavailability assays[J]. Plant and Soil, 2013, 369(1/2): 531-541. |
[65] |
Pérez A L, Anderson K A. Soil-diffusive gradient in thin films partition coefficients estimate metal bioavailability to crops at fertilized field sites[J]. Environmental Toxicology and Chemistry, 2009, 28(10): 2030-2037. DOI:10.1897/08-637.1 |
[66] |
Black A, McLaren R G, Reichman S M, et al. Evaluation of soil metal bioavailability estimates using two plant species(L. perenne and T. aestivum) grown in a range of agricultural soils treated with biosolids and metal salts[J]. Environmental Pollution, 2011, 159(6): 1523-1535. DOI:10.1016/j.envpol.2011.03.004 |
[67] |
Tandy S, Mundus S, Yngvesson J, et al. The use of DGT for prediction of plant available copper, zinc and phosphorus in agricultural soils[J]. Plant and Soil, 2011, 346(1): 167-180. |
[68] |
Duquène L, Vandenhove H, Tack F, et al. Diffusive gradient in thin films(DGT) compared with soil solution and labile uranium fraction for predicting uranium bioavailability to ryegrass[J]. Journal of Environmental Radioactivity, 2010, 101(2): 140-147. DOI:10.1016/j.jenvrad.2009.09.007 |
[69] |
Liu J, Feng X, Qiu G, et al. Prediction of methyl mercury uptake by rice plants(Oryza sativa L.) using the diffusive gradient in thin films technique[J]. Environmental Science & Technology, 2012, 46(20): 11013-11020. |
[70] |
李亚青, 万晨洁, 魏天娇, 等. 基于梯度扩散薄膜技术的脱水污泥中重金属有效性的原位分析[J]. 南京大学学报(自然科学), 2017, 53(2): 227-237. LI Ya-qing, WAN Chen-jie, WEI Tian-jiao, et al. In situ analysis of heavy metal availability in dewatered sewage sludge based on DGT technique[J]. Journal of Nanjing University(Natural Sciences), 2017, 53(2): 227-237. |