文章信息
- 程方圆, 周学永, 张家琛, 鲁伶兰, 马锦明
- CHENG Fang-yuan, ZHOU Xue-yong, ZHANG Jia-chen, LU Ling-lan, MA Jin-ming
- 阳离子对Bt蛋白在矿物表面吸附动力学的影响
- Effects of cations on the adsorption kinetics of Bt protein on mineral surfaces
- 农业环境科学学报, 2017, 36(9): 1844-1849
- Journal of Agro-Environment Science, 2017, 36(9): 1844-1849
- http://dx.doi.org/10.11654/jaes.2017-0172
文章历史
- 收稿日期: 2017-02-16
2. 天津市农药研究所, 天津 300400
2. Tianjin Institute of Pesticides, Tianjin 300400, China
苏云金杆菌(Bacillus thuringiensis,Bt)是一种广泛存在于土壤中的革兰氏阳性菌,在其芽孢形成过程中产生杀虫晶体蛋白[1]。自1987年Vaeck等[2]首次报道转Bt基因抗虫植物以来,转Bt基因棉花[3]、玉米[4]和茄子[5]相继实现商业化生产。我国自1997年开始种植Bt棉花,随着规模的不断扩大,目前种植面积已达370万hm2。近年来Bt杨树也开始种植,2015年栽培已达543 hm2[6]。
随着Bt作物种植面积的不断扩大,其环境安全性问题日益引起关注,Bt蛋白通过根系分泌、秸秆还田和花粉飘落[7]等方式释放到土壤,并迅速被矿物颗粒吸附[8]。一旦吸附完成,就能对微生物和酶的降解产生抗性,使持效期显著延长。由于Bt蛋白在土壤矿物表面吸附迅速,有关其动力学研究很少。刘洁等[9]研究了蒙脱石等四种矿物对Bt蛋白吸附的动力学,发现矿物性质、温度、pH对动力学特征有重要影响。近年的研究表明,除了温度、pH、矿物类型之外,离子强度也是影响Bt蛋白吸附的重要因素。刘宁等[10]研究了钠、钾离子对Bt蛋白在蒙脱石和凹凸棒石表面吸附和解吸的影响,证实钠、钾离子对Bt蛋白吸附有促进作用,且钾的影响稍高于钠。Fu等[11]研究了离子强度和倍半氧化物对Bt蛋白吸附的影响,证实在低浓度范围内( < 10 mmol·kg-1)钠、钙离子均对Bt蛋白吸附有促进作用。然而Sander等[12]则观察到了相反结果,在pH>6的条件下,Bt蛋白在土壤有机质上的吸附随着离子强度的降低而增加。
截至目前,离子强度对Bt蛋白吸附动力学的影响尚无文献报道。本文选取蒙脱石、高岭石、凹凸棒石和碳酸钙四种不同类型的矿物,研究Na+、K+、Mg2+、Ca2+四种阳离子对Bt蛋白吸附动力学的影响,并通过Bt蛋白、矿物表面电位变化对影响机制做出探讨,为环境风险评价提供实验依据。
1 材料与方法 1.1 实验材料Bt库斯塔克亚种原粉由华中农业大学微生物农药国家工程中心提供,蒙脱石由浙江丰虹粘土化工有限公司提供,凹凸棒石由江苏省盱眙县华丰油田钻井液用材料厂提供,高岭石购自上海五四化学试剂有限公司,超细碳酸钙由山西芮城华纳纳米材料有限公司提供。氯化钠、氯化钾、氯化镁和氯化钙均为分析纯。
1.2 Bt蛋白提取Bt蛋白的提取按照文献[10]进行。
1.3 金属阳离子对Bt蛋白吸附动力学的影响Bt蛋白用Tris缓冲液(0.01 mol·L-1,pH7.2)溶解,离心去除沉淀,取少量样品测定吸光度值,然后按照标准曲线计算其浓度。矿物预先配制成水悬液,吸附前离心管中预先加入金属盐溶液,并使其最终浓度为1 mmol·L-1。Bt蛋白和矿物按质量比1:5加入离心管中,在25 ℃条件下进行振荡吸附,分别在1、2、4、6、8、10、20、30、60、100 min进行高速离心(15 000 r·min-1,15 min),取上清液在280 nm测定吸光度,利用标准曲线计算Bt蛋白浓度,计算吸附量。同时做矿物空白和不添加金属离子的动力学实验。
1.4 金属阳离子对Bt蛋白和矿物表面电位的影响将Bt蛋白用Tris缓冲液配成0.2 mg·mL-1溶液,依次加入0、0.25、0.5、1.0、1.5、2.0 mmol·L-1金属盐溶液,混合后放置1 h,用电泳淌度仪(Nano-ZS90,Malvern Instruments,UK)测定Bt蛋白Zeta电位,用于确定合适的离子添加浓度[13]。矿物粉体分别用蒸馏水配成水悬液(1 mg·mL-1),然后与1 mmol·L-1金属盐溶液混合放置1 h,用电泳淌度仪测定Zeta电位。
1.5 Bt蛋白标准曲线的测定Bt蛋白配成0.3 mg·mL-1溶液,依次稀释为0.225、0.187 5、0.15、0.112 5、0.075、0.037 5 mg·mL-1,以Tris缓冲液作对照,在280 nm处测定吸光度。以横轴为Bt蛋白浓度、纵轴为吸光度值绘制标准曲线。
1.6 动力学模型 1.6.1 一级动力学模型[14]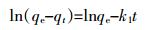
式中:qe是吸附达到最终平衡时的最大吸附量,mg·mg-1;qt是在任意时刻测定的吸附量,mg·mg-1;k1是吸附平衡常数,min-1。
用ln(qe-qt)对t作图得到直线,利用斜率可以求出k1,利用截距可以计算理论吸附量。
1.6.2 二级动力学模型[14]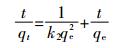
式中:k2是二级吸附平衡常数,mg·mg-1·min-1。
利用t/qt对t作图得到直线,利用斜率可以求出理论吸附量,再用截距计算k2。
1.6.3 内扩散模型[14]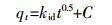
式中:kid是内扩散速率常数,mg·min-0.5。
用qt对t0.5作图得到直线,利用斜率可以求出kid。
1.6.4 Elovich模型[15]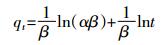
式中:α、β均为吸附常数。
用qt对lnt作图得到直线。
1.7 统计分析运用SPSS软件对吸附数据进行统计分析,并比较差异显著性。
2 结果与分析 2.1 金属阳离子对Bt蛋白在蒙脱石表面吸附动力学的影响Na+、K+、Mg2+、Ca2+对Bt蛋白在蒙脱石表面吸附动力学的影响如图 1所示。吸附体系不添加金属离子时,Bt蛋白吸附平衡时间为20 min;当添加1 mmol·L-1 Na+或K+时,吸附速率较无离子添加相差不大,经30 min吸附趋于平衡。当添加1 mmol·L-1 Mg2+或Ca2+时,吸附速率急剧增加,且Ca2+对Bt蛋白吸附的促进程度超过Mg2+。统计分析结果表明:Ca2+、Mg2+均对Bt蛋白吸附有极显著影响,且二者之间存在显著差异(P < 0.01);Na+、K+对Bt蛋白吸附有显著影响,但二者之间差异不显著(P >0.05)。
![]() |
图 1 金属阳离子对Bt蛋白在蒙脱石表面吸附动力学影响 Figure 1 Effects of metal cations on the adsorption kinetics of Bt protein on montmorillonite |
Na+、K+、Mg2+、Ca2+对Bt蛋白在凹凸棒石表面吸附动力学影响如图 2所示。吸附体系不添加金属离子时,Bt蛋白在凹凸棒石上的吸附特性与在蒙脱石上相似,20 min吸附即趋于平衡。当添加1 mmol·L-1 Na+或K+时,吸附速率较无离子添加时提高,且K+的提高程度大于Na+。当添加1 mmol·L-1 Mg2+或Ca2+时,2 min后吸附速率分别增加6.3倍和7.0倍,且Ca2+的促进作用大于Mg2+。统计分析结果表明:Ca2+、Mg2+均对Bt蛋白吸附有极显著影响(P < 0.01),且二者之间差异显著;K+对Bt蛋白吸附有显著影响,且与Na+之间存在显著性差异;Na+对Bt蛋白吸附无显著影响(P >0.05)。
![]() |
图 2 金属阳离子对Bt蛋白在凹凸棒石表面吸附动力学影响 Figure 2 Effects of metal cations on the adsorption kinetics of Bt protein on attapulgite |
Na+、K+、Mg2+、Ca2+对Bt蛋白在高岭石表面吸附动力学影响如图 3所示。吸附体系不添加金属离子时,Bt蛋白在高岭石表面的吸附60 min后趋于平衡,平衡时间较蒙脱石、凹凸棒石延长。当添加1 mmol·L-1 Na2+或K+离子时,初始吸附速率较不添加金属离子明显加快,平衡时间缩短至30 min,但Na+、K+之间差别不大。当添加1 mmol·L-1 Mg2+时,2 min后吸附速率增加15.9倍,30 min后趋于平衡。Ca2+对Bt蛋白吸附的促进作用大于Mg2+,与不添加金属离子相比,2 min后吸附速率增加22.7倍,20 min出现短暂的平衡后,至60 min时吸附又有小量增加。统计分析结果表明:Ca2+对Bt蛋白吸附有极显著影响(P < 0.01),Mg2+有显著影响(P < 0.05),Na+、K+无显著影响。
![]() |
图 3 金属阳离子对Bt蛋白在高岭石表面吸附动力学影响 Figure 3 Effects of metal cations on the adsorption kinetics of Bt protein on kaolinite |
Na+、K+、Mg2+、Ca2+对Bt蛋白在碳酸钙表面吸附动力学影响如图 4所示。吸附体系不添加金属离子时,Bt蛋白在碳酸钙表面的吸附20 min趋于平衡。当添加1 mmol·L-1 Na+时,吸附速率较无离子添加时加快,30 min吸附达到平衡,吸附速率提高1.5倍。当添加1 mmol·L-1 K+时,吸附速率较Na+添加进一步加快,至30 min吸附达到平衡时,吸附速率提高1.9倍。当添加1 mmol·L-1 Mg2+时,吸附速率较一价离子添加明显加快,30 min后吸附速率提高3.3倍。Ca2+对Bt蛋白吸附的影响明显超过Mg2+,前10 min吸附速率急剧增加,此后呈缓慢增加趋势,至吸附实验结束时,仍未出现明显的平衡拐点。统计分析结果表明:Na+对Bt蛋白吸附有显著影响,而K+、Mg2+、Ca2+三种离子则有极显著影响(P < 0.01),且彼此之间差异显著(P < 0.05)。
![]() |
图 4 金属阳离子对Bt蛋白在碳酸钙表面吸附动力学影响 Figure 4 Effect of metal cations on the adsorption kinetics of Bt protein on calcium carbonate |
Bt蛋白在四种矿物表面的吸附数据经过一级动力学模型拟合,R2在0.710 8~0.987 6之间(表 1),四种金属离子的影响顺序为:Ca2+>Mg2+>K+>Na+。依据相关性的知识判断,该模型基本能够反映Bt蛋白的吸附动力学特性[14]。但模型计算得出的平衡吸附量与实测值出入较大,故该模型拟合效果并不理想。
二级动力学模型拟合的R2在0.995 1~0.999 9之间(表 2)。该模型不仅相关系数高,而且由模型计算得出的平衡吸附量与实测值非常接近,故拟合效果理想。内扩散及Elovich模型的动力学模型拟合结果见表 3。内扩散模型的R2在0.619 4~0.898 0之间,相关性较低,表明该模型拟合效果不够理想;而Elovich模型的R2在0.914 1~0.982 9之间,相关性较高,表明该模型拟合效果比较理想。
![]() |
Na+、K+、Mg2+、Ca2+浓度对Bt蛋白表面电位影响如图 5所示。随着金属阳离子浓度提高,Bt蛋白表面电位受一价离子影响较小,其中K+的影响较Na+稍高。二价离子对Bt蛋白表面电位影响较大,离子浓度达到0.25 mmol·L-1时,Bt蛋白表面电位急剧升高,但当浓度超过0.5 mmol·L-1时,变化趋于平缓。总体比较,Ca2+的影响程度高于Mg2+。
![]() |
图 5 不同浓度金属阳离子在Bt杀虫蛋白表面的电位 Figure 5 The potential of metal cations at different concentrations on the surfaces of Bt protein |
凹凸棒石、高岭石、蒙脱石、碳酸钙在水溶液中表面电位均为负值,添加金属离子(1 mmol·L-1)后,表面电位均有不同程度的提高(图 6),四种金属离子对矿物表面电位的影响有着一致的规律性,即:Ca2+>Mg2+>K+>Na+。
![]() |
图 6 金属离子对矿物表面电位影响 Figure 6 Effect of metal cations on the surface potential of mineral |
金属离子促进Bt蛋白在矿物表面吸附的作用,是与矿物表面Zeta电位升高、胶体粒子团聚以及金属离子与Bt蛋白之间的螯合作用有关。首先,Bt蛋白等电点在5.5左右[10],在吸附条件下(pH7.2),Bt蛋白和矿物表面均带负电荷,因此Bt蛋白分子与矿物粒子之间存在着电荷斥力。随着金属离子浓度的提高,Bt蛋白和矿物表面的负电荷均朝着绝对值减小的方向变化,导致斥力减小,吸附量增加。土壤胶体双电层厚度与金属离子价态的关系如古依-查普曼(Gouy-Chapman)模型所示[16]:
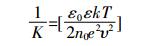
式中:1/K为扩散双电层厚度,ε0为真空介电常数,ε为水的介电常数,k为玻尔兹曼常数,T为绝对温度,n0为离子浓度,e为单位电荷,υ为阳离子价态数。
当其他条件不变时,阳离子价态越高,扩散双电层厚度越小,表面电势绝对值越低。对于同价离子而言,它们对胶体粒子Zeta电势的影响与离子水合半径有关。Na+、K+、Mg2+、Ca2+水合离子半径分别为0.358、0.331、0.428、0.412 nm[17],水合离子半径越大,扩散双电层厚度越大,Zeta电势绝对值越大。因Na+水合半径高于K+,Mg2+水合半径高于Ca2+,故Na+对Bt蛋白的促进作用低于K+,Mg2+对Bt蛋白的促进作用低于Ca2+。金属离子促进矿物吸附Bt蛋白的第二个原因,是矿物胶体粒子结构重排[18]。随着金属盐浓度的增加,矿物粒子双电层结构受到一定程度破坏,胶体粒子开始出现团聚现象,对Bt蛋白实行包裹或产生新的凹陷吸附位点,导致吸附量增加。此外,金属离子能够争夺Bt蛋白分子表面水化膜中的水分子,使Bt蛋白分子更易与矿物粒子接触,促进吸附发生。第三个原因则是钙镁二价金属离子可以与Bt蛋白形成螯合键,同时金属离子也容易被矿物吸附,从而使矿物与Bt蛋白之间形成盐桥[19],导致吸附量增加。
4 结论Na+、K+、Mg2+、Ca2+对Bt蛋白在矿物表面吸附动力学有一定影响,添加金属离子后,Bt蛋白吸附量增大,金属阳离子作用强弱依次为:Ca2+>Mg2+>K+>Na+。Bt蛋白吸附动力学可用二级动力学模型和Elovich模型来描述,其中二级动力学模型拟合结果最好,由该模型计算得出的平衡吸附量与实测值吻合;Elovich模型R2>0.914 1,也能够较好描述动力学特征。一级动力学模型R2在0.710 8~0.987 6之间,相关系数分布范围太宽,且由模型计算得出的平衡吸附量与实测值差异较大,故不适合用于动力学特征描述;内扩散模型相关系数低(R2>0.619 4),不建议在Bt蛋白吸附动力学中使用。
[1] |
Jouzani G S, Valijanian E, Sharafi R. Bacillus thuringiensis:A successful insecticide with new environmental features and tidings[J]. Applied Microbiology and Biotechnology, 2017, 101(7): 2691-2711. DOI:10.1007/s00253-017-8175-y |
[2] |
Vaeck M A, Reynaerts H, Hofte J, et al. Transgenic plants protected from insect attack[J]. Nature, 1987, 328: 33-37. DOI:10.1038/328033a0 |
[3] |
Yang Y, Li Y, Wu Y. Current status of insecticide resistance in Helicoverpa armigera after 15 years of Bt cotton planting in China[J]. Journal of Economic Entomology, 2013, 106(1): 375-381. DOI:10.1603/EC12286 |
[4] |
Etzold-Maxwell J L, Meinke L J, Gray M E, et al. Effect of Bt maize and soil insecticides on yield, injury, and rootworm survival:Implications for resistance management[J]. Journal of Economic Entomology, 2013, 106(5): 1941-1951. DOI:10.1603/EC13216 |
[5] |
Chakraborty T. Release of Bt-brinjal in bangladesh[J]. Economic and Political Weekly, 2014, 49(6): 24-26. |
[6] |
JamesC. 2015年全球生物技术/转基因作物商业化发展态势[J]. 中国生物工程杂志, 2016, 36(4): 1-11. James C. The global biotech/GM crops commercialization development situation in 2015[J]. China Biotechnology, 2016, 36(4): 1-11. |
[7] |
Strain K E, Lydy M J. The fate and transport of the Cry1Ab protein in an agricultural field and laboratory aquatic microcosms[J]. Chemosphere, 2015, 132: 94-100. DOI:10.1016/j.chemosphere.2015.03.005 |
[8] |
Stotzky G. Persistence and biological activity in soil of insecticidal proteins from Bacillus thuringiensis and of bacterial DNA bound on clays and humic acids[J]. Journal of Environmental Quality, 2000, 29(3): 691-705. |
[9] |
刘洁, 胡红青, 李慧姝, 等. Bt蛋白在不同矿物上的吸附动力学及其影响因素研究[J]. 土壤学报, 2010, 47(4): 786-789. LIU Jie, HU Hong-qing, LI Hui-shu, et al. Kinetics of adsorption of the toxin of Bacillus thuringiensis on minerals and its affecting factors[J]. Acta Pedologica Sinica, 2010, 47(4): 786-789. DOI:10.11766/trxb2010470428 |
[10] |
刘宁, 周学永, 董庆洁, 等. 钠、钾离子对Bt蛋白在蒙脱石和凹凸棒土表面吸附与解吸的影响[J]. 农业环境科学学报, 2012, 31(4): 786-790. LIU Ning, ZHOU Xue-yong, DONG Qing-jie, et al. Effects of sodium and potassium ion on the adsorption and desorption of Bt protein by montmorillonite and attapulgite[J]. Journal of Agro-Environment Science, 2012, 31(4): 786-790. |
[11] |
Fu Q L, Peng Y W, Huang T, et al. Effects of ionic strength and sesquioxides on adsorption of toxin of Bacillus thuringiensis subsp. kurstaki on soils[J]. Pedosphere, 2012, 22(1): 96-102. DOI:10.1016/S1002-0160(11)60195-1 |
[12] |
Sander M, Tomaszewski J E, Madliger M, et al. Adsorption of insecticidal Cry1Ab protein to humic substances:1. Experimental approach and mechanistic aspects[J]. Environmental Science & Technology, 2012, 46(18): 9923-9931. |
[13] |
Wang S Y, Chen K M, Li L, et al. Binding between proteins and cationic spherical polyelectrolyte brushes:Effect of pH, ionic strength, and stoichiometry[J]. Biomacromolecules, 2013, 14(3): 818-827. DOI:10.1021/bm301865g |
[14] |
Zaghouane-Boudiaf H, Boutahala M. Adsorption of 2, 4, 5-trichlo-rophenol by organo-montmorillonites from aqueous solutions:Kinetics and equilibrium studies[J]. Chemical Engineering Journal, 2011, 170(1): 120-126. DOI:10.1016/j.cej.2011.03.039 |
[15] |
Chien S H, Clayton W R. Application of Elovich equation to the kinetics of phosphate release and sorption in soils[J]. Soils Science Society of American Journal, 1980, 44(2): 265-268. DOI:10.2136/sssaj1980.03615995004400020013x |
[16] |
Yukselen Y, Kama A. Zeta potential of kaolinite in the presence of alkali, alkaline earth and hydrolyzable metal ion[J]. Water, Air, and Soil Pollution, 2003, 145(1/2/3/4): 155-168. |
[17] |
Nightingale Jr E R. Phenomenological theory of ion solvation:Effective radii of hydrated ions[J]. The Journal of Physical Chemistry, 1959, 63(9): 1381-1387. DOI:10.1021/j150579a011 |
[18] |
Cione A P P, Schmitt C C, Neumann M G, et al. The effect of added salt on the aggregation of clay particles[J]. Journal of Colloid and Interface Science, 2000, 226(2): 205-209. DOI:10.1006/jcis.2000.6812 |
[19] |
Muchaonyerwa P, Chevallier T, Pantani O L, et al. Adsorption of the pesticidal toxin from Bacillus thuringiensis subsp. tenebrionis on tropical soils and their particle-size fractions[J]. Geoderma, 2006, 133(3/4): 244-257. |